I have been involved in atomic and electronic structure
calculations, developing and employing various state-of-art packages
based on the formulations such as Density Functional Theory, Hartree
Fock Theory, Configuration Interaction method and Solvation
techniques throughout my research career.
Below are the details of various ongoing as well as past projects. Click on the titles to know more.
New theory for Magnetism in Random Alloys
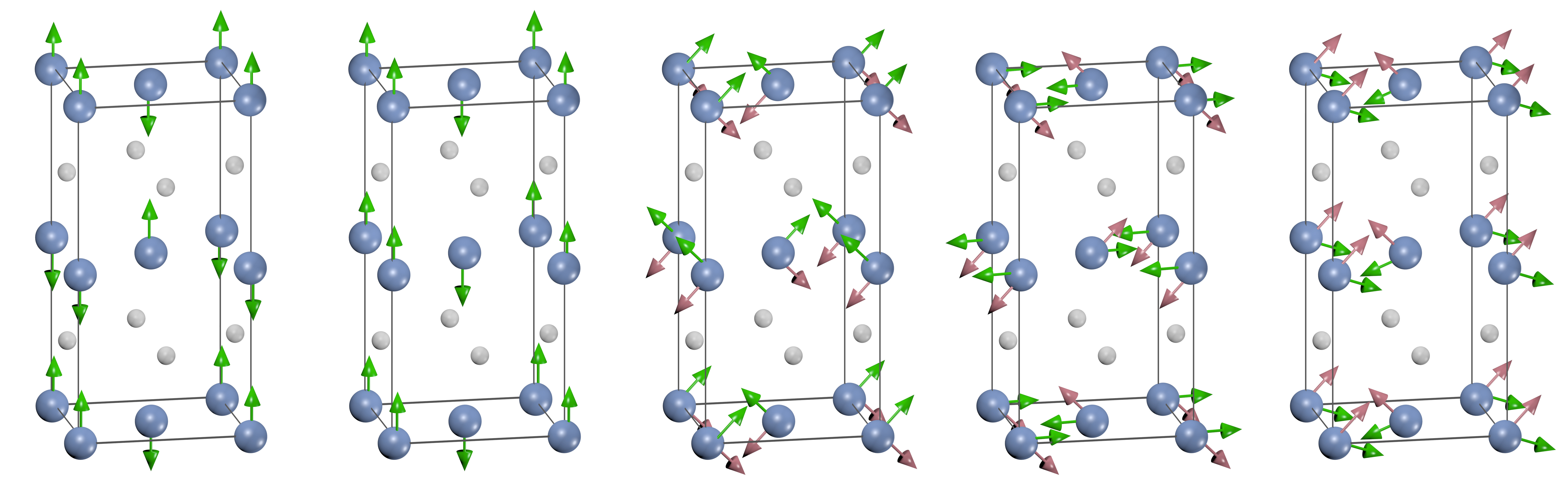
The Fe
1-xMn
xPt system exhibits five
magnetic structures, including a ferromagnetic phase, two
antiferromagnetic phases, and two noncollinear ones [1]. The
latter are characterized by superpositions of orthogonal magnetic
amplitudes at two commensurate magnetic wave vectors. Here we
study the magnetic interaction in this system and construct the
magnetic phase diagram based on total energy calculations within
the vector-DLM (disordered local moment) method representing
partially ordered magnetic states in the coherent potential
approximation (CPA). This method has been implemented within the
linear muffin-tin orbital basis. The calculated total energies
are fitted to polynomial functions of the relevant order
parameters (magnetization amplitudes at the relevant wave vectors
for the two components), which are subsequently used to evaluate
the free energy in the mean-field approximation. The interaction
between spatially orthogonal amplitudes is evaluated by
noncollinear total energy calculations for representative alloy
configurations. The phase diagram is constructed by minimizing
the free energy at each concentration and temperature. The
structure of the resulting phase diagram agrees with neutron
diffraction measurements, demonstrating the applicability of the
method to the studies of magnetic phase diagrams of intermetallic
alloys with competing ferromagnetic and antiferromagnetic
interactions.
[1] A. Z. Menshikov et al., J. Magn. Magn.
Mater. 65, 159 (1987).
Published in : Phys. Rev. Lett. 115, 057203 (2015)
Graphene and its derivatives
GraphAne - the hydrogenated graphene has been subject of interest lately. I worked on a few problems based on hydrogenation of graphene.
In the latest density functional study, we
demonstrate that single-side-hydrogenated graphene
(termed as
SSHGraphene) is a
semiconductor with an indirect band gap of 1.35 eV,
which is in between the gapless graph ene and wide
band-gap
graphane and
surprisingly close to silicon. We show that its
electronic structure and lattice characteristics are
substantially different from those of graphene,
graphone, or
graphane. The
lattice parameter and C-C bond length are found to be
lengthened by
15% of those of
graphene. Our binding-energy analysis confirms that
such a single-sided hydrogenation leads to
energetically stable material, making it a promising
candidate as an organic semiconductor.
Phys. Rev. B 84, 041402(R) (2011)
(Rapid Communications) 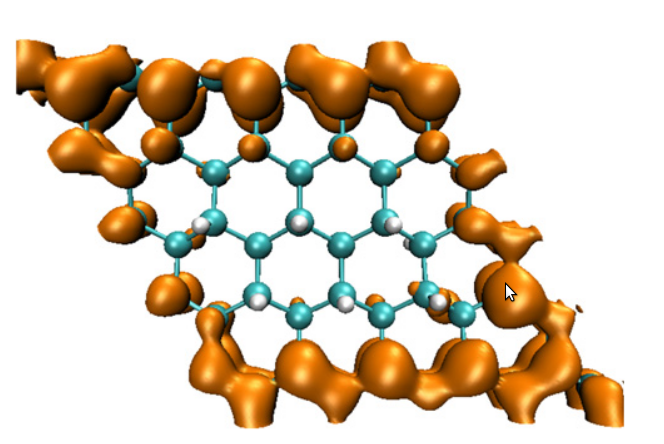
Earlier, we have probed the transformation of
graphene upon hydrogenation to graphane within the
framework of density functional theory. By analysing
the electronic structure for 18 different hydrogen
concentrations, we brought out some novel features of
this transition. Our results showed that the
hydrogenation favored clustered configurations
leading to the formation of compact islands. The
analysis of the charge densities and electron
localization functions (ELF) indicated that, as
hydrogen coverage increases, the semi-metal turns
into a metal, (showing a delocalized charge density,)
then transforms into an insulator. The metallic phase
was spatially inhomogeneous in the sense it contained
islands of insulating regions formed by hydrogenated
carbon atoms and metallic channels formed by
contiguous bare carbon atoms. It turned out that it
is possible to pattern the graphene sheet to tune the
electronic structure. For example, removal of
hydrogen atoms along the diagonal of the unit cell,
yielding an armchair pattern at the edge, gave rise
to a bandgap of 1.4 eV. We also showed that a weak
ferromagnetic state exists even for a large hydrogen
coverage whenever there was a sublattice imbalance in
the presence of an odd number of hydrogen atoms.
J. Phys.: Condens. Matter
22 465502,(2010) This work is
featured in “IOP Select November-2010”
In first problem we carried out ab initio electronic structure calculations on
graphane having single
and double vacancy defects. Our
analysis of the density of states reveals that such vacancies induce the
mid-gap states and modify the band gap. The induced states are due to
the unpaired electrons on carbon atoms surrounding the vacancy.
Interestingly, the placement and the number of such states is found to be
sensitive to the distance between the vacancies. It turns out that
such vacancies also induce a local magnetic moment.
J. Phys. Chem. C, 2009, 113 (50), pp 21063–21067
Proton hopping in Organometallic complex
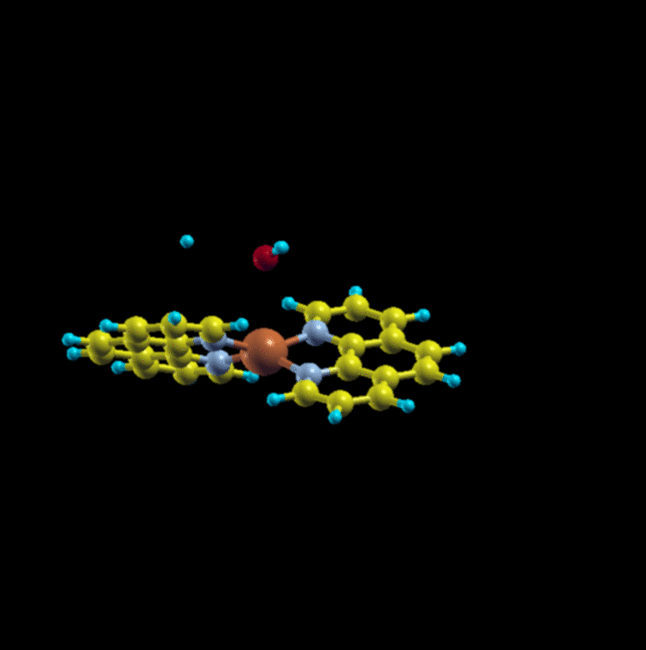
Using ab-inito molecular dynamics as well as Nudge Elastic Band simulations we examine the transfer of proton across a complex Organometallic compound. The compound synthesized by the experimental group at the Department of Chemistry shows the promising conductivity as a function of humidity. With the aid of ab-initio calculations we compute the activation energy along with the electronic structure calculations. The theoretical as well as computational results are in excellent agreement with each other.
Paper in communication
Materials under extreme conditions
Materials under high pressure and temperatures are of great interest,
especially in astrophysical applications. Among terrestrial and
extraterrestrial environments, it is quite common to have materials under
extreme physical conditions. It is known that the matter inside the mantle of
the Earth can be under more than 100 GPa pressure and is at temperatures about
900 C. Extraterrestrial environment offers even more exotic situations. For
example even at the surface of the Venus, temperature and pressure can reach as
high as 467 C and 9.3 MPa. It is a daunting task to know the exact nature
of the surface experimentally, because, it is difficult to simulate such
extreme situations in the laboratory and most of the astrophysical objects
are still beyond thorough observations.
We employ evolutionary algorithm coupled with state of the art density
functional theory, to study the crystal structure under extreme conditions that
mimics the extreme astrophysical situations, mainly Venus. The exact nature of
the surface of Venus is still under intense investigation and theoretical
predictions are warranted.
Ab-initio studies of Ferroelectric materials
Lead zirconate titanate (PZT), a ceramic perovskite material is used as a
component in ultra- sound transducers, ceramic capacitors, STM/AFM actuators,
FRAM chips and sensors. PZT has also been used in the manufacture of ceramic
resonators for reference timing in electronic circuitry. PZT is usually not
used in its pure form but doped with either acceptors to create anion vacancies
or with donors to create cation vacancies. Acceptor doping creates hard PZT and
donor doping creates soft PZT, which are differentiated based on their
piezoelectric constants. These piezoelectric constants are proportional to the
polarization i.e. the electric field generated per unit of mechanical stress.
Usually soft PZT has a higher piezoelectric constant but larger losses in
material whereas hard PZT has lower piezoelectric constant and lower losses in
material.
With this view, we are interested in performing first principles density
functional theory (DFT) based calculations on undoped and doped PZT materials.
So far most of my research work has been using density functional
theory. However one of problems with density functional theory is that the
number of atoms handled in the theory is seriously restricted by the
availability of the computational resources. Hence when it comes to
handling the mesoscopic systems like complex solute-solvent systems the
treatment by other methods are more useful. In my current work I
collaborate with experimental group who are interested in finding out
the optimum size of certain nanocrystals in given a solvent. We address
this issue by a method called 3D-Reference Index Site Model (RISM).
3D-RISM is based on integral equations and calculates the solvation
energies by solvent distribution. Our results are in excellent agreement
with the experiments and it provides in depth profiles of the
distribution of the molecules around the nanocrystals. I am fortunate to
be a part of the group which has done a fundamental contribution to
the RISM theory.
We developed a technique to decrease memory requirements when solving the
integral equations of three-dimensional (3D) molecular theory of solvation,
a.k.a. 3D reference interaction site model (3D-RISM), using the modified direct
inversion in the iterative subspace (MDIIS) numerical method of generalized
minimal residual type. The latter provides robust convergence, in particular,
for charged systems and electrolyte solutions with strong associative effects
for which damped iterations do not converge. The MDIIS solver (typically, with
2 × 10 iterative vectors of argument and residual for fast convergence) treats
the solute excluded volume (core), while handling the solvation shells in the
3D box with two vectors coupled with MDIIS iteratively and incorporating the
electrostatic asymptotics outside the box analytically. For solvated systems
from small to large macromolecules and solid–liquid interfaces, this results in
6- to 16-fold memory reduction and corresponding CPU load decrease in MDIIS. We
illustrated the new technique on solvated systems of chemical and biomolecular
relevance with different dimensionality, both in ambient water and aqueous
electrolyte solution, by solving the 3D-RISM equations with the
Kovalenko–Hirata (KH) closure, and the hypernetted chain (HNC) closure where
convergent. This core–shell-asymptotics technique coupling MDIIS for the
excluded volume core with iteration of the solvation shells converges as
efficiently as MDIIS for the whole 3D box and yields the solvation structure
and thermodynamics without loss of accuracy. Although being of benefit for
solutes of any size, this memory reduction becomes critical in 3D-RISM
calculations for large solvated systems, such as macromolecules in solution
with ions, ligands, and other cofactors.
Journal of Computational Chemistry
Volume 33, Issue 17, pages 1478–1494, 30 June 2012